Draft Report of the
AEGEAN SEA WORKSHOP
Rhodes, Greece, October 8-10, 2002
Sponsored by the Office of Naval
Research
and Office of Naval Research
International Field Office
Report prepared by the Workshop
Organizing Committee:
Sarantis
Sofianos (1)
William Johns (2)
Alexandros Lascaratos (1)
Stephen Murray (3)
Donald Olson (2)
Alexander Theocharis (4)
(1) Department
of Applied Physics
University of Athens
University Campus, Build PHYS-5
Athens, 15784, Greece
(2) Rosenstiel
School of Marine and Atmospheric Science
University of Miami
4600 Rickenbacker Causeway
Miami, FL 33149, USA
(3) Office of
Naval Research
800 N
Quincy Street
Arlington, VA 22217-5660, USA
(4) National Center for Marine Research
Institute of Oceanography
P.O. Box 712
Anabyssos-Attiki, 19013, Greece
Contents
1. Introduction
2. Scientific Background
3. Important Scientific Questions
4. Recommendations for Future Work
5. Acknowledgments
Reference/Appendices
A workshop was held, on October,
8-10, 2002, at the National Center for Marine Research / Hydrobiological Station of Rhodes, Greece, on the topic "Aegean
Sea", sponsored by the U.S. Office of Naval Research and the
Office of Naval Research International Field Office.
The workshop
focused on primarily on the physical oceanography and air-sea interaction of
the Aegean Sea but also considered biophysical interactions in the region. The
central objectives of the workshop can be summarized as:
a.
Discuss the present level of understanding
of the physical oceanography and related disciplines of the Aegean Sea. Exchange
information between scientists with experience in the region and/or similar
cases, which can help to summarize the known facts and the outstanding
questions of the dominant mechanisms governing the Aegean Sea.
b.
Identify important topics for future study
in the Aegean Sea on different time scales (synoptic, seasonal and climatic) and
possible approaches (observations and modeling). Establish collaborations
between scientists and institutes to help the planning of collaborative
international projects.
Over thirty
scientists from four countries (Greece, USA, Turkey and Germany) participated in the workshop, including theoreticians,
observationalists, and numerical modelers (Appendix 1). During the first day of
the workshop thirteen invited talks were presented on various topics of the
dynamics governing the Aegean Sea circulation, exchange with adjacent basins, air-sea
interaction, as well as the biophysical characteristics of the region (Appendix
2). Following the scientific presentations the workshop was organized in three
working group sessions. The central theme of Session 1 was the review of the
dynamics and circulation of the Aegean Sea, and the participants broke into two groups focusing on: (A.1)
short to seasonal time scales and (A.2) interannual/climatic variability. In
Session 2, the discussion focused on the priorities for future research, and
the participants broke into the same two groups: (B.1) short to seasonal time
scales and (B.2) interannual/climatic variability). Finally, the third working
group session dealt with forming recommendations for future work and suggesting
possible approaches to this research, in two working groups: (C.1)
observational and (C.2) modeling approaches. All these groups were not sharply
separated, and there was considerable overlap within each group as well as
interaction between the groups (in the plenary sessions and by participation in
different groups).
This report
contains a summary of the discussion that took place during the three days of
the Aegean Sea Workshop. Section 2 highlights the principle features of the Aegean Sea circulation
and exchange with the atmosphere and the adjacent basins, in various time
scales. Results from recent experiments
(not yet published) are also briefly presented. Section 3 presents the most
important scientific questions concerning the dynamics of region, as were
summarized by the group discussion sessions, followed by a summary and
recommendations for future research in Section 4.
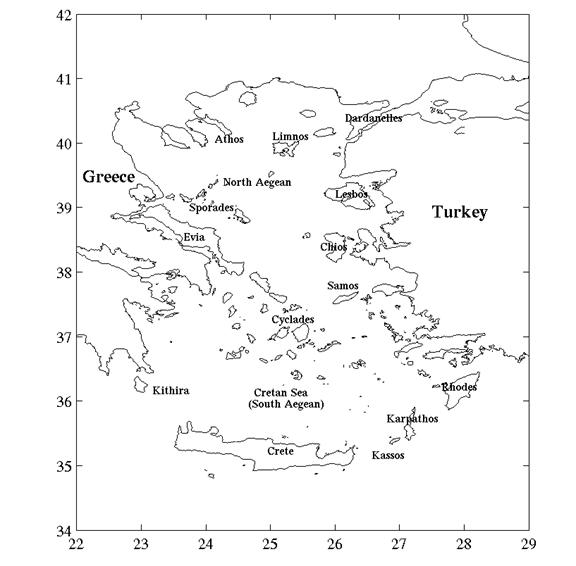
Figure 1. Major islands and basins in
the Aegean Sea region
2.1 Geographical
Characteristics
The Aegean Sea
is one of the four major basins of the Eastern Mediterranean Sea,
covering an area of 240,000 km2. It is situated in the northeastern Mediterranean,
to the northeast of the Ionian Sea and to northwest of
the Levantine Sea,
bounded to the north and west by the Greek mainland, to the east by the Turkish
coasts and to the south by the islands of the Cretan Arc. The Aegean sea
is connected with the Marmara and Black
Seas through the strait of Dardanelles
(average width 3.5 km; sill depth
70 m). At its southern end it is connected with the Levantine and Ionian Seas
through a series of six straits (the Cretan Arc Straits), including (from east
to west): the Rhodes Strait (width 17 km; sill depth 350 m), the Karpathos
Strait (width 43 km; sill depth 850 m), the Kassos Strait (width 67 km; sill
depth 1000 m), the Antikithira Strait (width 32 km; sill depth 700 m), the
Kithira Strait (width 33 km; sill depth 160 m), and the Elafonissos Strait (width
11 km; sill depth 180 m).
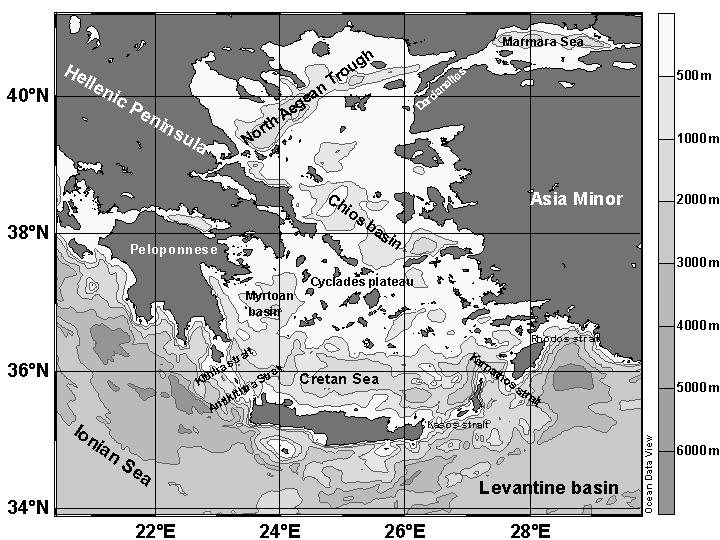
Figure 2. Basic topographic features of the Aegean Sea region.
The
Aegean Sea coastline is very irregular and its
topographic structure very complicated. There are over 3,000 islands of various
sizes scattered all over the basin. Three deep basins exist: the North Aegean Trough
oriented in the WSW-ENE direction (including the N. Sporades, Athos, Limnos and
Saros basins) in the northern Aegean with maximum depths up to 1,500 m, the
Chios basin in the central Aegean
with maximum depth of 1,100 m, and the Cretan basin in the southern
Aegean, which is the largest and deepest one, with maximum depths of 2,500 m.
The Chios basin is bounded to the south by the extensive
Cyclades plateau and sills (with depths that do not
exceed the 350 m), which is identified in the literature as the limit between
the north and south Aegean.
2.2 Atmospheric Forcing
The wind field over the Aegean
Sea is strong and its direction is largely controlled by
orography. The prevailing winds, on the annual mean basis, are of the northern
sector. The seasonal wind stress distribution is presented in Fig 1 (May,
1982). The seasonal cycle of the wind field is associated with the strength and
the persistence of the northerly winds and has two maxima, one during the
winter (December - February) and a second one during summer (July - August).
During winter the wind is primarily from the north (during this season, the Aegean
Sea occasionally experiences strong southerlies, (Katsoulis,
1970)) bringing cold and dry air through the various river valleys of the Balkan
peninsula. During summer the wind field is dominated by the
Etesian winds, which are northerlies, dry, and can reach gale force. Moderate
diurnal sea breezes are present along the coasts during
summer.
The evaporation rate over the
Aegean Sea is around 1.3 - 1.5
m/year (da Silva et al., 1994; Jakovides et al., 1989) with maximum values
during February and August. The annual mean net heat flux is estimated at 26
W/m2 (Poulos et al., 1997), which implies that the Aegean
Sea is, on the average, losing heat through its surface. This loss
is balanced by advection of the warm Levantine waters through the Cretan Arc
straits (while the heat exchange with the Black Sea is
negligible (Tolmazin, 1985)).
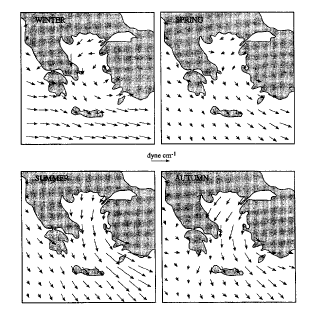
Figure 3. Seasonal pattern of the wind stress (after May, 1982)
The Aegean Sea
receives the colder and fresher Black Sea Water (BSW) through the Bosphorus, Marmara
Sea and the Dardanelles
strait. Below that layer there exists an outflow of the more saline Aegean Sea
waters. The BSW has a rate of 1,257 km3/year while the
Aegean Sea waters a rate of 957 km3/year (Unluata et al., 1990). The Aegean
Sea also receives the freshwater outflows from various rivers
along the Greek and Turkish coasts. The annual mean evaporation rate (E)
exceeds the sum of precipitation (P) and river runoff (R) (0.5 m/year and 0.11
m/year, respectively) (Poulos et al., 1997). However, if the influence of the
BSW is taken into account, then the water balance of the Aegean Sea
is negative (E-P-R-BSW<0). The excess volume of water per unit area has been
calculated between 0.7 and 1.4 m/year (Bethoux and Gentili, 1994; Poulos et
al., 1997). The water budget of the region is compensated by the advection of
waters through the Cretan Arc straits.
2.3 Circulation and
water mass formation
Despite the progress in direct
observations and modeling efforts during the last two
decades (many of these studies were presented during the presentation session
of the workshop, see Appendix 2.), the circulation of the Aegean Sea
is yet far from being well defined and understood. The circulation pattern that emerges from observations is
rather complex and variable. This is due to many factors, such as the distribution of
the various island chains and straits, the irregular bottom topography, the
seasonal variability of the atmospheric forcing, the presence of strong meteorological
events that may alter the local circulation patterns, and the presence of many
different water masses.
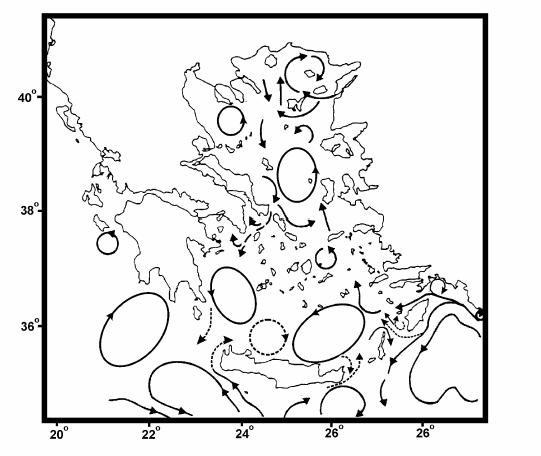
Figure
4.
Schematic of the major upper layer
circulation of the Aegean Sea (after Lykousis et al.,
2002)
If we attempt to summarize the
known circulation characteristics (from various cruises covering parts of the region,
recent drifter deployment, and modeling techniques), there seems to be a
general cyclonic circulation in the Aegean Sea. However,
the most active dynamic features are the mesoscale cyclonic and anticyclonic
eddies, which can extend to several Rossby radii of deformation (around O(10 km)). The spatial and temporal
variability of these features is not really known. Some of these features
appear to be permanent (i.e. the cyclonic eddy in the south Chios
basin), while others have a transient character. The dynamics responsible for
the formation (and decay) of these features is not clear. The wind field, the
thermohaline forcing, and the interaction of these features with the very
complex topography are suggested as the mechanisms involved in the
generation and spatiotemporal variability some of the identified eddies.
The most characteristic feature
of the circulation pattern in the north Aegean is the
surface outflow of the brackish BSW from Dardanelles,
which creates a front with the ambient saltier waters of Levantine origin.
These waters have a general westward movement and then southward, being modulated by the
presence of mesoscale eddies (Zodiatis, 1994). They cross the various passages towards
the westward side of the Cyclades plateau and, after
experiencing strong mixing, they appear at the western side of the Cretan
Sea in the southern Aegean.
The dynamics of the BSW plume and its interaction with the irregular coastline
and topography (islands, bays, straits) is not well understood even though it
plays an important role in the biochemical processes of the region. The basic
water masses in the northern Aegean are three: the BSW,
the highly saline and warm waters of Levantine origin (LSW and LIW), and the
very dense deep waters that fills the bottom of the various
sub-basins. These deep waters are formed locally (both shelf and open sea convection
processes have been hypothesized as the mechanisms involved in the North Aegean deep water
formation) with strong interannual variability (Theocharis and Georgopoulos,
1993; Zervakis et al., 2000). It should be noted that the local formation of
extremely dense waters in the North Aegean takes place despite the fact that the
latter is much more stratified than the South Aegean, due to the presence of
the BSW layer at the surface. Several studies (Plakhin, 1971, 1972; Zervakis et al., 2000)
have commented on the role as an insulator of the surface layer of BSW in the North Aegean,
and on the impication it has in deep water formation processes. Thus, the variability
of buoyancy input from the Dardanelles could be a significant contributor to the
thermohaline circulation of the Aegean.
The southern Aegean (Cretan
Sea) and the Cretan Arc straits
have been more intensely investigated during recent international projects
(Georgopoulos et al., 1989, 2000; Roether and Schlitzer, 1991; Theocharis et
al., 1993, 1999). This investigation revealed
intense mesoscale variability, including transient and/or recurrent cyclonic
and anticyclonic eddies (Fig 4). The exchange between the Cretan Sea and the
Levantine and Ionian basins is very complex, presenting strong seasonal and
interannual variability, as well as interaction with local circulation features
(Asia Minor Current, Mirtoan/West Cretan Cyclone, East Cretan Cyclone, the
Ierapetra Anticyclone and the Rhodes Gyre) (Kontoyiannis et al., 1999). Direct
measurements revealed a persistent deep outflow of Cretan Deep Water (CDW) (sq>29.2)
with an annual mean of ~0.6 Sv, through the Antikithira and Kassos Straits at
depths below 400 m and 500 m, during 1994-95, respectively. However, during
1987-95 the CDW outflow presents large variability with peak values exceeding
1 Sv (Theocharis et al., 1999). The
typical water mass structure of the Cretan
Sea (until the dramatic change of
the late 1980s, see below), included in the upper layer the saline surface
waters of Levantine origin (LSW; S~39 psu) and the less saline (S<38.9)
surface waters of Black Sea origin (BSW). The inflow of LSW seems to occur mostly
during the warm period of the year and enters the SE Aegean
through the eastern Cretan Arc Straits,
while the BSW comes from the North Aegean and
affects mainly the Mirtoan basin
and western Cretan Sea.
Frequently there are also intrusions through the Cretan Arc Straits of the less
saline subsurface water of Atlantic origin, the so-called Atlantic
Water (AW) originating from the Ionian Sea. Below the
upper layer and down to the bottom, two water masses were distinguished with
similar characteristics, but slightly denser (σθ up to
29.16) than those of the Levantine Intermediate Water (LIW). This relatively
dense Cretan water was observed to overflow occasionally through the Cretan
straits and contribute to the water masses below the LIW in the Eastern
Mediterranean (Schlitzer et al., 1993; Malanotte-Rizzoli et al., 1997).
Observations after the early 1990s revealed a dramatically
different structure of the Cretan Sea
water column. Extremely dense (σθ
> 29.2) and very saline (S >39 psu) water, of local origin, started
filling the deep Cretan basin and overflowing through the sills of the Cretan
Arc straits (Roether et al., 1996; Kontoyiannis et al., 1999; Theocharis et al.,
1999; Theocharis et al., 1999a). Due to its high density, the Cretan Deep Water (CDW) displaced water
from the deepest parts of the Levantine and Ionian basins of the Eastern
Mediterranean becoming a major contributor of deep and bottom water in the Eastern
Mediterranean. So, the present state of the Cretan Sea includes
three distinctive water masses in the intermediate and deep layers: (i) the Cretan Intermediate Water (CIW), formed locally and
located between 50 and 250 dbar, with physical characteristics similar to those
of the LIW, but still warmer and slightly saltier, (ii) the so-called
Transitional Mediterranean Water (TMW), a mixture between the LIW and the
Eastern Mediterranean Deep Water of Adriatic origin found a depths up to 700 dbar, coming from the
mid-depths of the Eastern Mediterranean through the eastern and western Cretan
Arc Straits, and, (iii) the CDW in the deepest/ bottom parts of the basin
(800-2500 dbar), formed by open sea convection and/or in the surrounding shelf
areas (Theocharis et al., 1999; Lykousis et al., 2002). This structure is not
static but seems to undergo considerable trends and changes. For example, the
CDW volume in the Cretan Sea
has declined recently, most probably due to lack of very dense water
formation.
Figure
5.
Internal waves on both
sides of Crete (picture taken from
"Oceanography from the space shuttle", ed. D. C. Honhart,
UCAR-ONR, 1989)
The coupling between the north and south Aegean
through the Cyclades plateau (and other topographic
barriers, such as the Sporades island chain) is one
of the most unexplored issues of the Aegean Sea
dynamics. Zervakis et al. (2000) suggested that during periods of massive dense water formation
in the North Aegean the north-south exchange is greatly enhanced and the thermohaline
circulation of the Aegean is accelerated. Water masses are exchanged through a very complex series of island
chains and straits of different sizes. Exchange mechanisms and enhanced mixing
processes that may occur in these regions have not been studied. Semi-diurnal
tidal currents in the Aegean Sea are of low magnitude in
comparison with the overall flow and, therefore, tidal forcing is unlikely to
play a major role in the mixing processes of the region. Information on
internal waves is very limited although energetic internal waves have been
identified in areas that are characterized by strong flows, complex vertical
structure and irregular topography. These
oscillations, especially their interaction with the bottom topography, may be
having significant effects on the stratification of the region (e.g. disrupt
the seasonal thermocline) (Velegrakis et al., 1999; Zervakis et al., 2003).
2.4 Interannual/Climatic Variability
The importance of Aegean Sea to the
thermohaline circulation of the Mediterranean Sea has
long been a controversial topic (Pollack, 1951; Wust, 1961; Miller, 1963;
El-Gindy and El-Din, 1986; Malanotte-Rizzoli and Hecht, 1988). Although several
investigators proposed that the Aegean Sea is
contributing to the Eastern Mediterranean Deep circulation, it has been
generally accepted that the Adriatic Sea was the major
source of Eastern Mediterranean Deep Water (EMDW). This picture changed
abruptly in the early 1990s, when hydrographic surveys revealed that the
circulation in the area was undergoing a major transition (Roether et al.,
1996; Theocharis et al., 1992), in which the Aegean
Sea deep waters became a dominant source for EMDW. The density of
the deep waters in the Cretan Sea
increased dramatically and very dense water (CDW) was able to outflow and sink
to the bottom of the Eastern Mediterranean Sea. It was
estimated that this event started at the late 1980s (as early as 1988) and that
the volume contribution of new water was at least 1 Sv, exceeding the Adriatic
source by a factor of three. This event was termed the Eastern Mediterranean
Transient (EMT) and has been the focus of several studies during recent years (Roether et al., 1996;
Theocharis et al., 1999; Klein et al., 1999; Lascaratos et al., 1999; Samuel et
al., 1999; Malanotte-Rizzoli et al., 1999; Zervakis et al.,
2000;
Wu et al., 2000; Boscolo and Bryden, 2001; Tsimplis and Josey, 2001; Stratford and Haines, 2002;
Tsimplis and Rixen, 2002; Theocharis et al., 2002).
Although all investigators agree that climatic transient is a combined effect
of temperature and salinity changes, there are several hypotheses proposed by
investigators for the causes of this climatic transient. Changes in the water
budget of the Aegean Sea (E-P, the role of BSW inflow
from Dardanelles, etc.), the presence of strong winters
in the late 1980s / early 1990s and changes in the circulation characteristics
in the Levantine Sea
which caused accumulation of salt in the basin are among the mechanisms
proposed as the causes of the EMT.
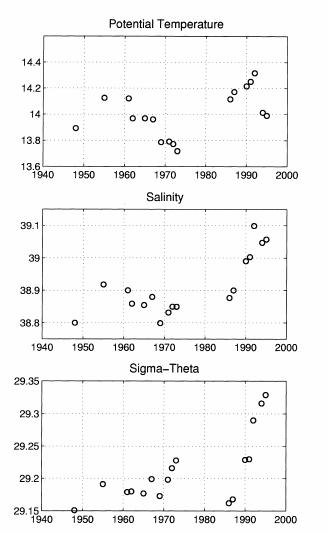
Figure 6. Time series of the annual mean potential temperature, salinity, and sigma-theta at 1000 m in the Cretan Sea (from Lascaratos et al., 1999)
Although the Aegean Sea
experiences strong interannual variability on different time scales, the EMT is
the strongest signal of climatic variability, and is most probably connected
with many different dynamical aspects of the circulation, water mass formation,
and air-sea interaction, including effects on the general circulation of the Mediterranean
Sea. There is clear evidence that the EMT is an ongoing process
(Lykousis et al., 2002), but the present and future status of the process, its
effects on the general circulation of the Mediterranean Sea,
and its connection with large scale climatic processes (e.g. NAO) remain
obscure. Finally, although we can identify several locations where there are
clear indications of dense water formation (including the North Aegean, the
Mirtoan Sea, the Cyclades plateau, and the Cretan Sea), the exact locations,
formation rates, mechanisms involved, and interannual variability are important
points in order to understand the role of the Aegean sea as part of the general
Mediterranean thermohaline system.
2.5 Biophysical
coupling
The
irregular coastline (gulfs, islands, straits) and the complex bottom topography
together with the complex hydrology and circulation in the Aegean Sea
lead to a great diversity of biotopes and strong spatial variability of the
pelagic communities. The Aegean Sea can be characterized
as an oligotrophic basin but one that shows strong north-south gradients in
plankton biomass and productivity (Fig 5). Recent observations suggest that a
microbial food web dominates in the North Aegean (in
which copepods are feeding mainly on protozoa), while a
multivorous food web (with copepods feeding mainly on on both protozoa and large phytoplankton) dominates in
the South Aegean (Lykousis et al., 2002). The North
Aegean is characterized as a special environment due to the
existence of important rivers and hydrological fronts, including the influence
of the BSW. This is also reflected to the higher trophic levels (fish and
benthos) of the North Aegean, where a more efficient
energy transfer through the pelagic food web is taking place.

Figure 7. Annual mean phytoplankton pigments distribution (from Siokou-Frangou, Workshop presentation)
Among the physical factors
playing an important role in the biochemical processes of the region are the
mesoscale variability of the circulation patterns, deep water formation, bottom
topography, BSW inflow, and river runoff. The atmospheric forcing and its
spatial and temporal variability are also important in establishing regions of
coastal upwelling and downwelling, which are mostly associated with the strong
Etesian winds. The temporal and spatial variability of the circulation in the Aegean
Sea is reflected in the biochemical processes. The simple picture
of the mean north-south gradient presented here is significantly obscured by
seasonal variability (e.g. seasonal variability of the BSW outflow and river
runoff) and mesoscale variability (e.g. there are also strong gradients of
biochemical processes within the same basin).
Recent advances in ecological modeling of the Aegean Sea
(Triantafyllou, 1999) have produced important tools that can help improve our
understanding of the physical and biochemical coupling of the region.
In this section we summarize the
basic scientific questions posed by the workshop participants as the most
interesting and/or unresolved aspects of the Aegean Sea
dynamics. The questions are broken into two sections, short time scales (up to
seasonal variability) and interannual time scales, following the group
discussion sessions that took place during the workshop.
3.1 Short time scales
- Satellite images show changes in surface glitter
caused by prevailing northerly winds blowing through gaps in the mostly
high islands of the Aegean archipelago.
How do the resulting patterns of surface wind stress around the
islands affect eddies, internal waves, and mixing?
- The Aegean is relatively
shallow compared to ocean basins and has many sills, ridges, and
islands. In addition, tidal
currents are much weaker than in the major ocean basins. How does the internal wave field change
near these bathymetric variations?
From shallow to deep water?
From islands to open water? How is the production of internal waves
in a region of complex bathymetry coupled to wind forcing?
- Owing to the shallow depths and short distances
between islands internal waves may be dissipated before they propagate
far, precluding establishment of a steady-state background spectrum. Is there a background internal wave
spectrum? Does the intensity of the
internal wave field vary with seasonal changes in wind stress?
- The Aegean contains strong
quasi-permanent eddies or gyres with horizontal scales smaller than typical
mesoscale eddies in the open ocean.
There are also several strong jets.
How do these eddies and jets modulate
mixing?
- What features of the circulation are permanent versus
seasonal, or of even shorter lifetime? What are the mechanisms involved in
the formation and/or decay of these features (e.g. wind versus
thermohaline forcing, topographic effects)? Why does there appear to be
increased eddy activity in summer?
- How do circulation features, boundary currents, jets
and eddies interact with straits of varying width and depth?
- Atmospheric circulation over the Aegean
is neither maritime nor continental.
How does the Planetary Boundary Layer change through the
archipelago? What effects do these
changes have on visibility? On
surface wind stress and buoyancy flux?
- How the wind stress pattern is affected by the presence of
complex orography and what is the wind shear effect on the exchange through the various straits?
- What are the patterns and dominant processes
affecting oceanic convection and restratification? How are these processes modulated by
islands, plateaux, and eddies?
- Where, at what rate, and when, are deep water masses
formed in the Aegean Sea? How does mixing across
the different plateaux and straits transform the water properties (e.g.,
what is the role of Cyclades plateau in the strong
physical and biological contrast between the North and South
Aegean)?
- How do the mixing patterns affect biological
production?
- What is the time history of the Dardanelles
outflow? What is the shape of the Dardanelles
plume? Does the plume break into
boluses or does it remain connected?
- What are the issues about underwater acoustics in the
Aegean and how do they result from the above
phenomena?
3.2 Interannual
Variability
Although there is already a
significant scientific discussion on the causes and role of EMT, there are
still a large number of questions concerning the evolution of this event, as
well as variability on other time scales. The discussion group focusing on the
priorities of the investigation of the interannual variability in the Aegean
Sea identified four basic groups of questions.
- What is the interannual variability of the Aegean
Sea and how this is coupled to the variability of the whole Mediterranean
Sea? Although EMT is a unique oceanic process that needs
special attention, variability on other time scales and periods should
also be focused in the region. Furthermore, the connection of EMT with
other climatic processes may help understand its causes and evolution.
- What are the driving factors of the interannual
variability in the Aegean Sea? What is the relative
importance of the air-sea heat fluxes, lateral fluxes (exchange between the
Aegean Sea and the adjacent basins through the Dardanelles
and the Cretan Arc straits), and freshwater budget (evaporation,
precipitation and river runoff)? What is the difference in the variability
between the North and South Aegean and how these
are coupled? It was also made clear from the group discussion that
monitoring the interannual variability of the region should include a
broader geographical area (e.g. Eastern Mediterranean,
Black Sea) since the interaction between basins and
feedback mechanisms are important in understanding the dynamics that drive
variability on long time scales.
- How does the Aegean Sea
respond to changes in the atmospheric forcing and interaction with the
adjacent basins? How and why the water mass formation processes differ in
the various sub-basins? What is the effect of long term variability on the
exchange between the Red Sea, the Black
Sea and the Eastern Mediterranean
basins? How do extreme events (e.g. very cold winters) affect the long
term variability of the Aegean Sea?
- What are the underlying dynamics of the EMT and its
relaxation time scales? Are we heading toward the "old" state of deep and
intermediate circulation in the Eastern Mediterranean
or will the relaxation state be different?
A strong consensus emerged
between the workshop participants that the Aegean Sea
can be a useful test basin for the
investigation of several oceanic processes since it incorporates many different scales
(several basin sizes, islands, straits, forcing mechanisms of different scales,
as well as oceanic processes of different spatial and temporal scales). Both
wind and thermohaline forcing is strong in the region. The tidal signal is weak
in the region and cannot mask the effects of atmospheric forcing and bottom
topography in regulating the mixing processes. The existence of strong
atmospheric and oceanic numerical model communities with well established
operational systems (Poseidon system, ALERMO/SKIRON system, NRL/NAVO system)
can also facilitate the understanding of
the Aegean Sea dynamics as well as process studies in
the region. Below we summarize recommendations for strategies and methods, as
were discussed in the working group sessions (observational and modeling
methods, approaches, and opportunities for collaboration).
4.1 Observations
Science issues can be divided
loosely into three categories: (1) Long-term monitoring to quantify variability
on interannual to interdecadal scales, (2) Exploratory efforts directed at
obtaining a baseline picture of variability in under-observed regions and (3)
Process studies focused on specific dynamical questions. Long-term monitoring
efforts have already begun (e.g. buoys of the NCMR Poseidon System), though
existing efforts are of limited scope, and the design of a more comprehensive,
cost-efficient systems would benefit from advances in understanding produced by
a intensive investigations of critical elements of the
Aegean Sea, Black Sea and Eastern
Mediterranean. Likewise, efforts to quantify and understand
long-timescale variability, along with the findings of exploratory programs,
will provide context and focus for investigations of critical dynamical
questions. As an initial step, participants highlighted the importance of
compiling and analyzing archived oceanographic and meteorological data to
generate basic statistical measures of variability. For example, simple metrics
such as the frequency, intensity and duration of strong wind events would be
useful for interpreting historical data and for planning future measurement
programs.
Several aspects of Aegean
oceanography were identified as requiring a baseline set of observations. At
long timescales, these focused on understanding interannual and interdecadal
variability, on general circulation studies focused on the northern basin, and
on characterization of deep circulation. Ideally, an extended (decades)
measurement program would document basin-wide variability in watermass
structure and circulation while attempting to understand the results in the context
of variations in both local and remote forcing. Financial constraints shape
strategies for achieving an extended program of in situ measurements. These
efforts must balance the need to achieve broad, basin-scale scope, sampled at
seasonal timescales, with the practical considerations needed to assure
continuous, funded operations for periods spanning ten or more years.
Discussions turned toward a suite of cost-efficient platforms and integrative
measurement techniques. The possibility of continuous SOLO/PALACE float
deployments, with deep parking depths designed to keep them from being carried
rapidly out of the Aegean, offers a promising avenue
that would benefit from the rapidly growing ARGO program. A float program could
characterize deep and intermediate circulation while providing basin-wide
hydrographic profiles. Surface drifters could be deployed to map surface
circulation and provide distributed meteorological measurements. Although
Lagrangian technologies are well suited for circulation studies, other methods
must be employed to monitor key straits and passages. Sparse moored arrays,
augmented with autonomous gliders executing cross-strait sections, offer a
cost-efficient system for making extended measurements at the fine lateral
scale necessary to resolve these flows. None of these approaches would permit
the regular sampling of nutrients and tracers, which can provide unique
insights into changing circulation patterns. Such sampling requires regular
occupation of a well defined array of hydrographic stations. The prospect
of recruiting and equipping voluntary observing vessels also offers a promising
avenue for making cost-efficient, sustained measurements. Numerous ferries ply
the Aegean throughout the year, many of which might be
equipped with ADCPs, hull mounted temperature and salinity sensors or, perhaps,
one of the low-cost moving vessel profiling systems presently under
development. Such vessels also offer possible platforms for making sustained
meteorological measurements (e.g. the SeaKeepers
program, which places meteorological sensors aboard recreational yachts).
However, there are serious difficulties that must be overcome to produce
high-quality meteorological measurements from voluntary observing platforms.
Accurate measurements of the full suite of fluxes are costly and require
greater care that is typical of voluntary platforms.
The Aegean
provides opportunities for a wide range of exploratory and process studies,
many of which might proceed as a single coordinated effort. Examples of this
approach include the recent ONR-funded Japan/East Sea program, which combined
basin-scale float and hydrographic programs with mesoscale studies of eddy and
front dynamics to advance our understanding of the dynamics governing
circulation within a semi-enclosed marginal sea. At a minimum, exploratory
efforts will likely be required to optimize the design of proposed process
studies. Strong, episodic wind and buoyancy forcing at extremely small (O(10 km)) lateral scales characterize
atmospheric forcing in several recent investigations of marginal seas. We have
only limited understanding of the dynamics governing the oceanic response to
forcing at scales similar to the deformation radius, though recent theoretical
and modeling studies suggest interesting mechanisms for frontogenesis and
subduction may be active under these conditions. The combination of intense,
small-scale forcing and complex bathymetry will severely impact internal wave
generation and propagation and produce spatial and temporal modulation of
mixing. Simultaneous investigations of the marine boundary layer would
contribute significantly toward advancing our understanding of many of these
problems. Although the specific design of any process study hinges on
foreknowledge of the interesting dynamics, such efforts in the Aegean
will likely share the need to sample at spatial scales on the order of
kilometers and time scales of days. For example, many of the straits will
require fine lateral resolution, as strait widths are often larger than the
deformation radii, admitting complex recirculations that must be resolved. Most
of the processes discussed by the working group are clearly three-dimensional,
placing additional demands on sampling methodologies. Long sampling periods
(obtained from moorings, floats, drifters, long-range AUVs or extended cruises)
or highly responsive sampling may be required to capture multiple realizations
of response to episodic forcing events. Specific methodologies and instruments
cannot be selected without first choosing a problem and designing an actual
measurement program. Nonetheless, technologies that the working group found
particularly intriguing included:
- HF radar for 3-D maps of surface currents. This
technology seemed well suited given the Aegean’s
many islands.
- Profiling moorings and ADCPs. Limited fishing
activity (relative to many other marginal seas) might ease operational
constraints on these platforms.
- Microstructure profiling for quantifying temporal and
spatial distribution of elevated mixing.
- Towed, undulating profilers to resolve synoptic,
small scale, three-dimensional variability.
- Traditional hydrographic/ADCP/bio-optical profiling
for large-scale coverage, in the context of already scheduled series of research/buoy-maintenance
cruises by cost efficient vessels, that cannot be
obtained using autonomous sensors.
- Floats and drifters, perhaps deployed in the context
of a basin-scale monitoring program, but used to augment specific process
studies.
- Long-range autonomous gliders. These could be
directed in real time to sample specific features, or used to augment
intensive investigations by repeatedly occupying sections of acting as
profiling moorings.
All of the investigations
envisaged would require combinations of these sampling technologies to
adequately resolve the processes of interest. Lastly, although the work group
discussed the potential value of including biological, chemical and bio-optical
sampling, details were not pursued.
4.2 Modeling
Atmospheric and oceanic modeling
of the Eastern Mediterranean, and especially the Aegean
Sea, has a long and successful history. There are several
"realistic" and "idealized" modeling studies covering parts of the Aegean
Sea, the whole basin, or a larger area including the Aegean
region, as well as operational/forecasting systems (Poseidon system,
ALERMO/SKIRON system, NRL/NAVO system). Despite the progress, there are several
limitations of the modeling systems, such as:
- The high complexity of bottom topography and
geography in the area points out the need for higher resolution
atmospheric modeling and generation of high-resolution forcing fields.
- There is lack of data for the Dardanelles
inflow, and its interannual variability. Also, the numerical treatment of
the inflow presents difficulties to be resolved.
- Subsurface measurements of velocity, temperature and
salinity that can be used as model constraints and provide means for
better parameterization of ocean models are very sparse in the Aegean
Sea. This is more important in areas of abrupt topographic
gradients (straits, islands, etc.).
- Data assimilation in the existing models (e.g.
TOPEX/Poseidon data) is very difficult due to the presence of the numerous
islands and islets.
Beyond improving the existing
numerical models, the Aegean Sea presents great
challenges to modeling studies of various problems. The variety of circulation
scales, topographic features, atmospheric and lateral forcing mechanisms
introduce many interesting cases where models can help to understand the
underlying dynamics while at the same time improving their own skill (e.g.
parameterization of mixing and dissipation). Improved modeling of mixing
processes is very important as supported by recent results (Spall, 2002, his
presentation in the Aegean Sea Workshop) that suggest the basin wide
circulation in semi-enclosed basins is largely affected by boundary mixing
processes. Some examples of important modeling issues and processes that were
discussed in the discussion group session are presented below:
- High resolution modeling studies to investigate the flow
response to sudden, small scale wind events and interaction with complex
topography and islands.
- Idealized and realistic modeling studies of the flow
dynamics through multiple islands connected by narrow sills.
- Investigation of deep water formation in the North
Aegean and Cretan Sea
in order to identify permanent and occasional sources. This will help in
the quantification of deep water production rates, and improve our
understanding of the spreading dynamics, and mixing processes.
- Model experiments on interannual time scales to study
the influence of freshwater sources on the Aegean
and larger (e.g. Mediterranean) scale.
- Modeling studies of plume dynamics (BSW inflow,
river runoff, etc.).
- Study the evolution of EMT and its relaxation time
scales under various scenarios.
- Investigation of the coupling between atmosphere and
ocean on interannual time scales.
- Modeling the Aegean Sea
ecosystem dynamics in various scales. Investigate the role of Saharan dust
in the region.
Support for the Aegean Sea
Workshop was provided by the Office of Naval Research and the Office of Naval
Research International Field office. The workshop was held at the
Hydrobiological Station of Rhodes, and the organizing committee wishes to thank
the National Center
for Marine Research, the Station's director Dr. A. Sioulas and all the staff of
the Station for providing a perfect environment for such a workshop. We would
like to thank the working group leaders and rapporteurs:
D. Olson and V. Kourafalou (A.1), W. Roether and K. Nittis (A.2), M. Gregg and H.
Kontoyiannis (B.1), E. Ozsoy and A. Mantziafou (B.2), C. Lee and N. Skliris
(C.1), and A. Lascaratos and J. Pullen (C.2).
Appendix 1. List of participants
Appendix 2. Presentations